If proteins are the workforce of the cell, then protein folding enables workers to assume the shapes needed to do their jobs. When the work is done, a special class of proteins known as AAA+ ATPases harness the energy of adenosine triphosphate (ATP) to unfold or remodel proteins.
NIEHS Distinguished Lecture Series speaker Tania Baker, Ph.D., from the Massachusetts Institute of Technology (MIT), has studied these specialized proteins for decades. As she told the virtual audience during her Oct. 19 talk, one question continues to puzzle her and others in the field: why does the cell need not one, but two types of AAA+ ATPases? Baker presented her current research exploring the advantages and disadvantages of those types of AAA+ ATPases.
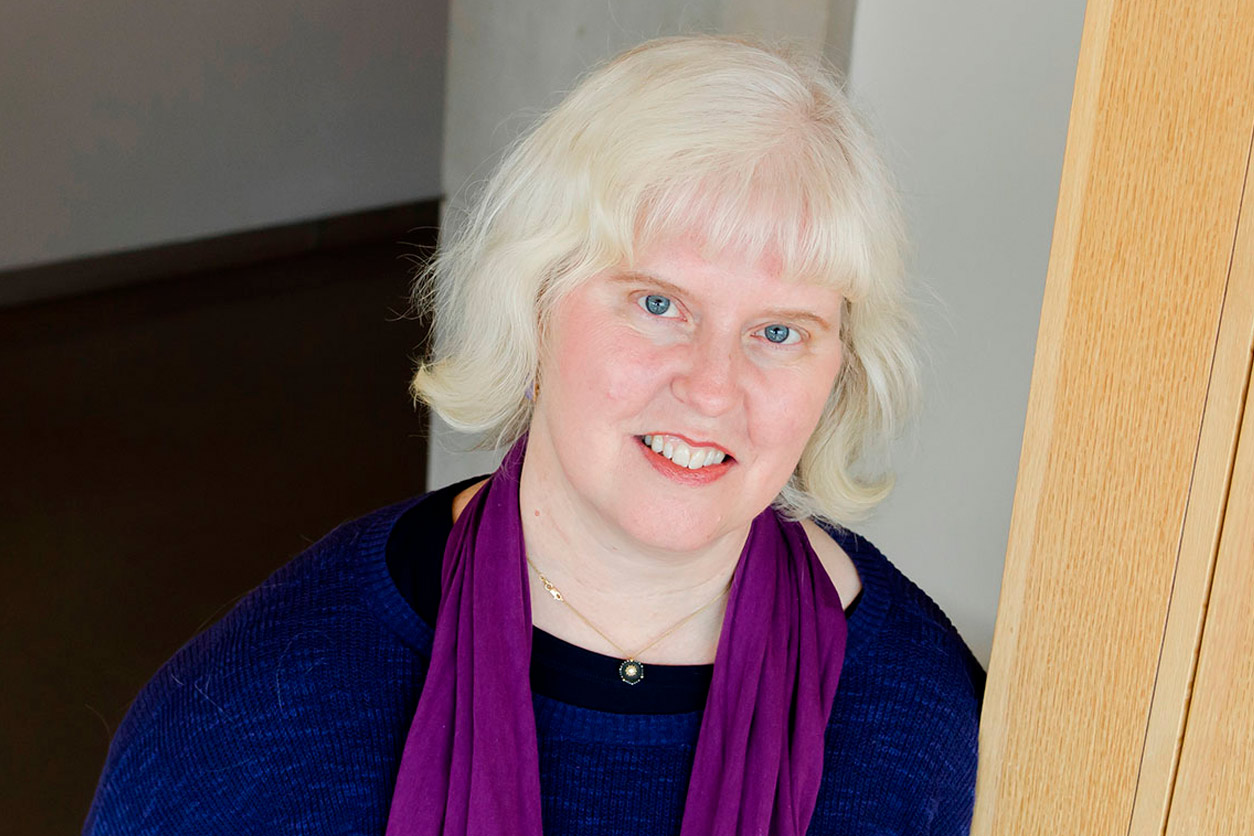
Robin Stanley, Ph.D., a Stadtman Investigator who leads the NIEHS Nucleolar Integrity Group, hosted the event. She explained that AAA+ ATPases are critical for regulating numerous cellular pathways, such as DNA replication, protein degradation, membrane fusion, and ribosome assembly.
“Baker's seminal work on AAA+ ATPases has implications for understanding bacterial antibiotic resistance, human mitochondrial metabolic disorders, and human diseases associated with protein misfolding,” she said.
Molecular motors
How well proteins fold and unfold can significantly affect their structure and function. According to Baker, AAA+ ATPases can form what is called an unfoldase motor. The term refers to the fact that the proteins assemble into a tight ring that uses ATP energy to shove folded proteins that pass through it into an unfolded single chain of amino acids.
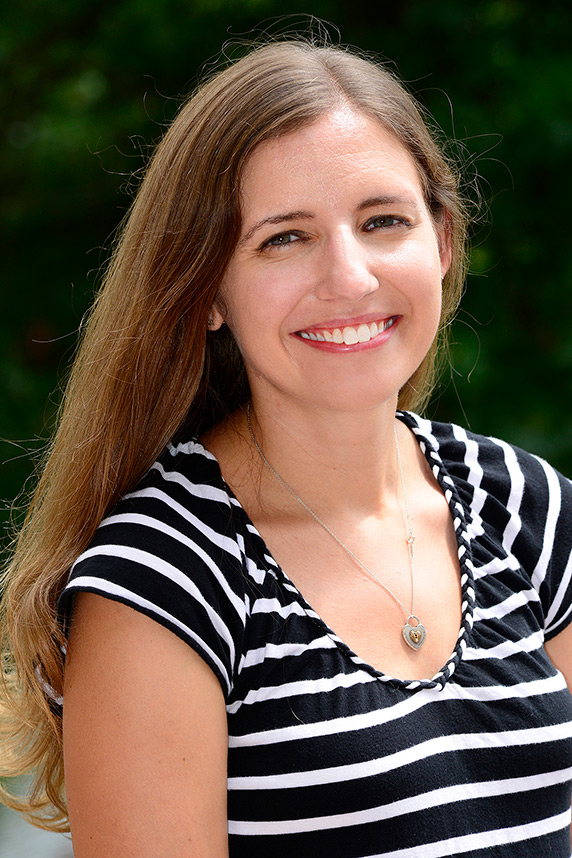
“However, whereas some of these motors have only one ring, others have two,” she said. “Why evolution has maintained both structures is not well understood.”
It takes two
To answer that question, Baker focused on a single-ring motor known as ClpX and a double-ring structure called ClpA. Her laboratory subjected both types to a litany of protein unfolding challenges and then compared their performance.
For example, when the researchers tested how well the enzymes unfolded a series of proteins, they found that both types exerted the same amount of power pulling the proteins through their pores. Yet, in the end, the double-ring configuration appeared to be faster and better than the single-ring one at unfolding many of the test proteins.
Baker said that having two rings, one stacked on top of the other, could create an advantage by creating more contacts and thus a better grip between the structure and the protein it is unfolding.
“You can think of it as a tug of war,” she explained. “If one team had resin on their hands and really gripped the rope well and the other team was equally strong but had just put Vaseline or hand lotion on their hands, then the resin team would make more efficient contacts with the rope and would probably win the tug of war.”
Evolution’s explanation
Baker believes that the double-ring configuration could also allow each ring to evolve more specialized functions. For instance, the top ring could be dedicated to binding and engaging proteins while the bottom one could focus on unfolding.
“This is an emerging picture of the double-ring AAA+ ATPase family members, although this work is still an ongoing area of research,” she said.
(Marla Broadfoot, Ph.D., is a contract writer for the NIEHS Office of Communications and Public Liaison.)