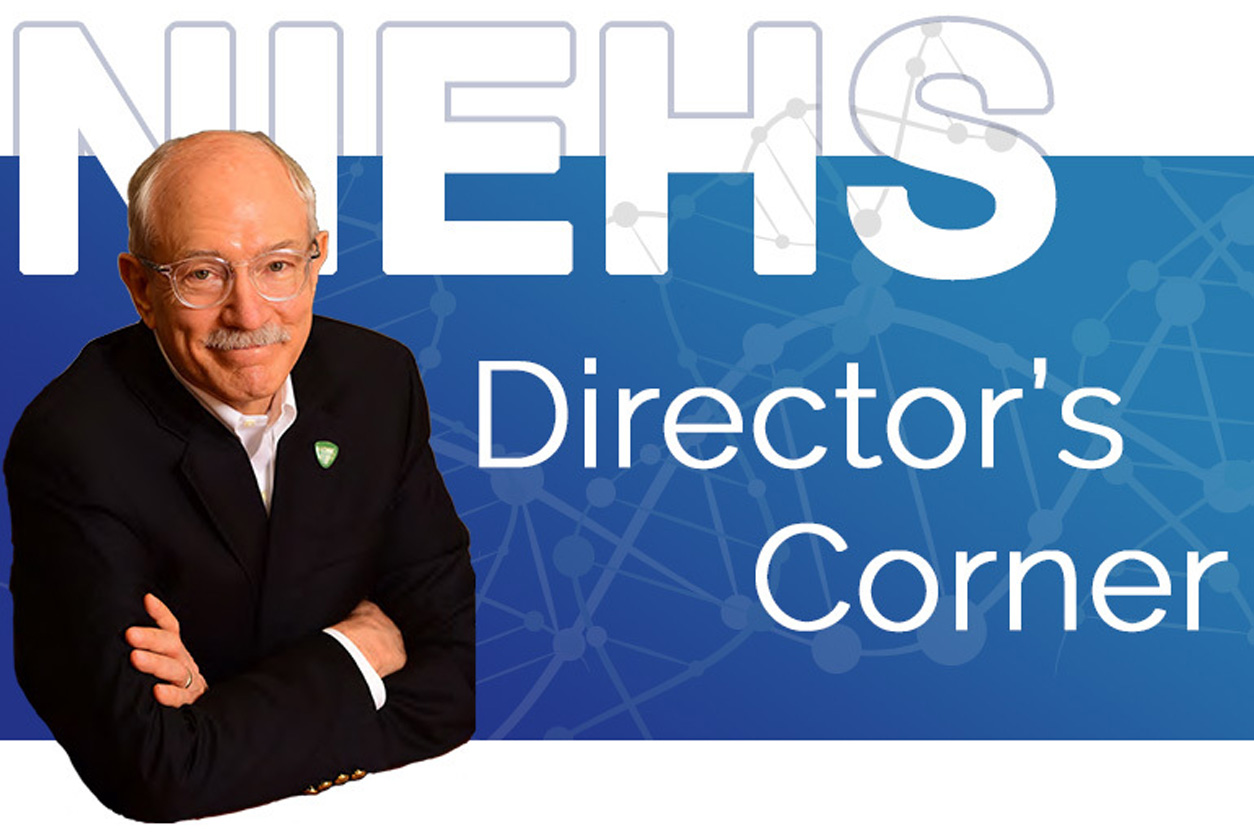
For decades, the field of medicine has focused largely on treating diseases after they develop. But what if we could identify risks and prevent illness long before symptoms appear? That’s the transformative vision behind precision environmental health (PEH) — a research framework that combines genetics, environmental exposure analysis, and data science to reveal both health risks at the individual level and opportunities for prevention.
To explore how PEH is reshaping scientific efforts and our understanding of health, I recently spoke with Andrea Baccarelli, M.D., Ph.D., a pioneering researcher and longtime NIEHS grantee. Dean of the faculty at the Harvard T.H. Chan School of Public Health and a member of the National Academy of Medicine, Baccarelli has spent his career uncovering how our exposures can influence health at the molecular level. His groundbreaking research into the ways environmental factors can modify gene expression without altering DNA, which scientists call epigenetic changes, has revealed that such changes can leave lasting biological “fingerprints.”
Those fingerprints act as a record of past exposures, from air pollution and lead to diet and exercise, providing vital clues about disease risk and even molecular aging. By analyzing these epigenetic marks, scientists can assess the exposome more robustly and better study how environmental factors can shape long-term health outcomes. The exposome refers to all of the exposures a person experiences in life and their corresponding biological effects.
During our conversation, Baccarelli shared his insights on epigenetics and exposomics as well as his exploration of extracellular vesicles — tiny messengers in the blood that may offer a noninvasive way to assess organ health. He also reflected on his career journey and what inspired his passion for uncovering the connections between the environment and human health.
Reframing our perspective on health
Rick Woychik: Can you share more about PEH and how it differs from precision medicine?
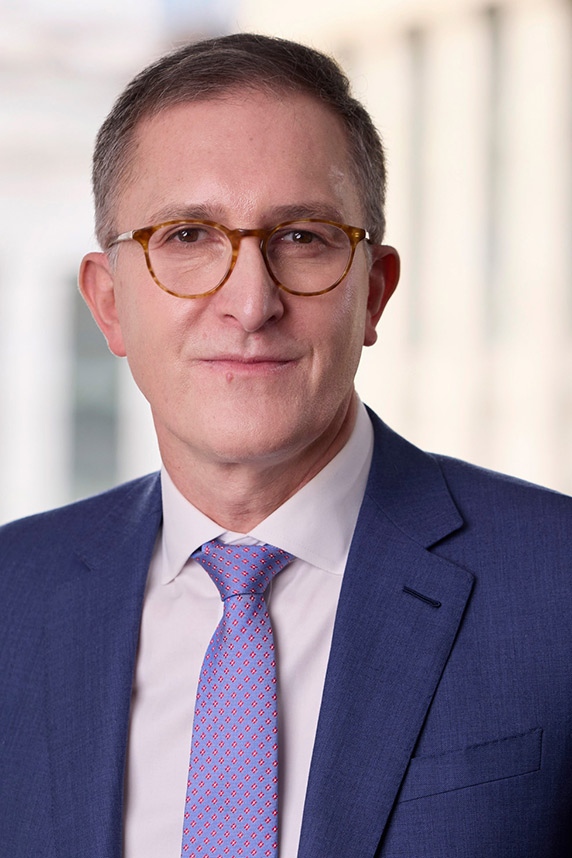
Andrea Baccarelli: Sure. PEH involves three major areas of knowledge: environmental exposures, genetics, including epigenetics, and data science. It is a research framework that also encompasses any type of omics, or molecular measures that reflect what happens in our bodies and cells, such as metabolomics, transcriptomics, and so forth.
Today, we have a great ability to assess our environmental exposures, understand what occurs within our bodies, and apply tools to effectively analyze all of this data. This puts us in position to identify individuals who, following certain exposures, may be more susceptible to disease or perhaps more likely to benefit from a particular diet, for example.
As for how PEH differs from precision medicine, I see precision environmental health as larger in scope. In fact, I think of precision medicine as a branch of precision environmental health. As a doctor, I’ve seen that health doesn’t only happen in the doctor’s office. In fact, the focus in that setting tends to be on disease — people are sick, and it’s our job to fix them. But true health develops during the months, years, and lifetime before someone gets a diagnosis — the times when we are best poised to intervene. So, a big part of PEH is helping individuals avoid disease altogether. That is far better than treating illness after it occurs.
That represents a major cultural shift in healthcare. In reality, what we call healthcare today is more like “disease care.” I’ve experienced this personally. After recovering from a virus that left me bedridden for three weeks, I asked my doctor how I could prevent it from happening again. His response was: “What’s the problem? You get a virus, we treat you, and you’re fine.” PEH involves a different perspective, focusing much more on prevention rather than treatment.
A more holistic look at exposures
RW: I think that’s great. As you mentioned, precision environmental health incorporates exposure analysis. In my view, it is critical for researchers to go beyond studying one exposure at a time and instead adopt the exposome framework. What are your thoughts on the exposome?
AB: The exposome has been a significant emphasis in environmental health over the past five years. Years ago, when I started my postdoctoral training and my first faculty position, each of us focused on one exposure at a time. There was an expert in particulate matter, another in dioxins, and so on. Each study addressed only a single exposure. However, we now recognize that our health is shaped by a combination of many exposures, both negative and positive.
Take the obesity epidemic as an example. Many chemicals can slow metabolism or increase appetite, but it’s not just one chemical — it’s a whole array of them, all contributing to shifts in weight. At the same time, factors like diet, access to healthy food, and opportunities for physical activity play crucial roles. For a single health outcome, such as body weight, there are hundreds of potential exposures, including chemicals, lifestyle factors, social influences, and so forth, all of which can tip the scales in one direction or another.
This is why the current emphasis on the exposome is so important. It offers a more holistic approach that helps us better identify what’s happening. Behind this approach is the understanding that exposures interact with one another. Exposure A alone and exposure B alone might not have the same effect as when they occur together, where their interaction could create a synergy that must be studied and understood.
Environment leaves “fingerprints” for scientists
RW: What tools do scientists have — or need to develop — to study the exposome?
AB: In terms of tools to study the exposome, the ability to measure hundreds or even thousands of chemicals simultaneously has advanced the field and encouraged us to move beyond the “one chemical at a time” framework. For example, here at Harvard, we are launching a new exposome lab that will use high-resolution metabolomics to measure up to 1,000 chemicals at once. It will also capture nutrients and the body’s metabolic responses. At the same time, data science will provide us with the opportunity to handle these large datasets and contextualize them.
In my lab, one of our contributions involves using the epigenome to “fingerprint” exposures. We are fortunate because the environment leaves numerous fingerprints on our cells, which we can study and analyze. This insight into the epigenome reveals the dynamic ways our bodies respond to environmental challenges and changes.
Every time there is a change in the environment around us, our body and cells react to it. Using omics technology, we can better understand these changes. Our objective is to create a unique fingerprint for each of the thousands of exposures we encounter, based on the cellular reactions they trigger. We have focused on DNA methylation, a type of epigenetic change that serves as a system through which our cells retain “memories” of past exposures.
One of the challenges with studying the exposome is its vast scope. It encompasses all exposures that affect our health, starting from conception and possibly even before conception. Some colleagues are exploring exposures that occurred in our parents' lives. However, studying exposures retrospectively is complex because research often begins at a specific point in an individual’s life. For instance, Dr. Manish Arora at Mount Sinai has developed a method to analyze exposures using teeth. By drilling into teeth, he can reconstruct exposure histories that span years, though this method typically focuses on one chemical at a time.
With the epigenome, we have shown that it can reveal the effects of exposures on our cells — and by extension, our bodies — years before a blood sample is taken. Algorithms and data-driven models are now enabling us to predict past exposures with remarkable accuracy. For example, there are algorithms that can identify smoking history or previous lead exposure.
Orchestrating gene expression
RW: Can you elaborate on the epigenome and its importance from a research perspective?
AB: Imagine a music book for a symphony or an opera. The book includes all the notes and notation, much like DNA is a code developed over time to carry genetic information. Just as a music score guides musicians to create a performance, DNA contains the instructions our bodies use to produce RNA and proteins. DNA is made of nucleotides, while a music score uses symbols to guide a performance.
Now, imagine the conductor’s music book on stage. It’s often filled with handwritten notes and markings that don’t alter the original score but help execute or express the music in a specific way. These annotations represent the epigenome — marks on DNA that don’t change its sequence but determine how it’s expressed. This is crucial because although we have about 20,000 genes in each cell, only about 2,000 —10% of them — are active at any given time.
Each cell type has a unique combination of active genes. For instance, brain cells express genes specific to the brain but not to muscles, while muscle cells express muscle-specific genes. These decisions are controlled by complex mechanisms, with epigenetics playing a central role in deciding which genes are active or inactive. The epigenome’s dynamic nature allows our bodies to adapt to environmental changes, keeping us healthy and functional.
What’s fascinating is that some epigenetic marks are written in ink, meaning they’re permanent. For example, during embryonic development, certain changes guide undifferentiated cells to become muscle, brain, or blood cells. Other marks are written in pencil, meaning they are easily erased or modified, allowing cells to respond to temporary changes like infections or stress. This dynamic system enables researchers to reconstruct past exposures and understand their long-term impacts on health. This will be critical to both exposomics and precision environmental health.
‘Liquid biopsies’ hold promise
RW: I understand that you have done exciting work analyzing extracellular vesicles, or EVs. Why are they so promising for environmental health and biomedical research?
AB: Extracellular vesicles are fascinating because they offer a way to study tissues that we can’t easily access through traditional methods. These tiny, membrane-bound vesicles are released by all cells and circulate in the blood. They carry molecular messages, like RNA, proteins, and lipids, that reflect the health and function of their cells of origin.
For instance, neurons in the brain release vesicles into the bloodstream, allowing us to study brain health using just a blood sample. Similarly, vesicles from lung cells or liver cells can provide insights into those organs. This noninvasive approach, often called a "liquid biopsy," has the potential to revolutionize how we monitor and understand organ health.
What excites me most is the ability to enrich samples for vesicles from specific cell types. In my lab, we’ve developed techniques to isolate vesicles from neurons, astrocytes, and other cells, which enables us to analyze environmental impacts on those tissues. For example, we can study how air pollution affects brain cells by analyzing neuron-derived vesicles from blood samples.
This field is still emerging, but it offers immense potential for both agnostic approaches — identifying biomarkers without predefined hypotheses — and hypothesis-driven studies that delve into specific biological mechanisms. EVs are essentially a previously hidden communication system between cells, and unlocking their secrets could transform both environmental health and biomedical research.
(Rick Woychik, Ph.D., directs NIEHS and the National Toxicology Program.)