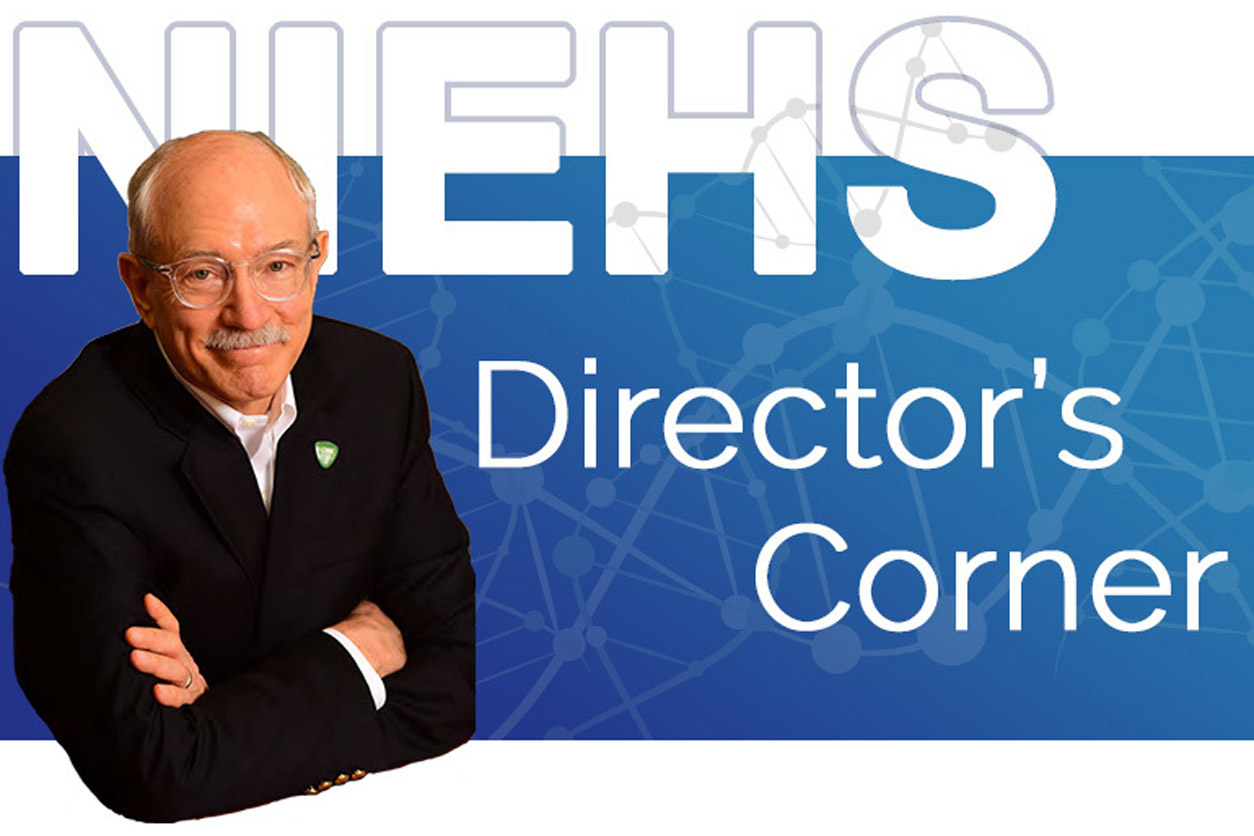
Environmental contamination is a pressing challenge in parts of the U.S. and in many places around the world, affecting ecosystems, wildlife, and human health. From legacy pollutants like PCBs (polychlorinated biphenyls) to emerging contaminants such as PFAS (per- and polyfluoroalkyl substances), the complexity and scale of exposures is often daunting. But within these challenges lies opportunity for innovation, where it is possible to harness scientific and engineering breakthroughs to clean up contamination and protect public health. Recently, I had the privilege of speaking with someone who has dedicated his career to doing just that.
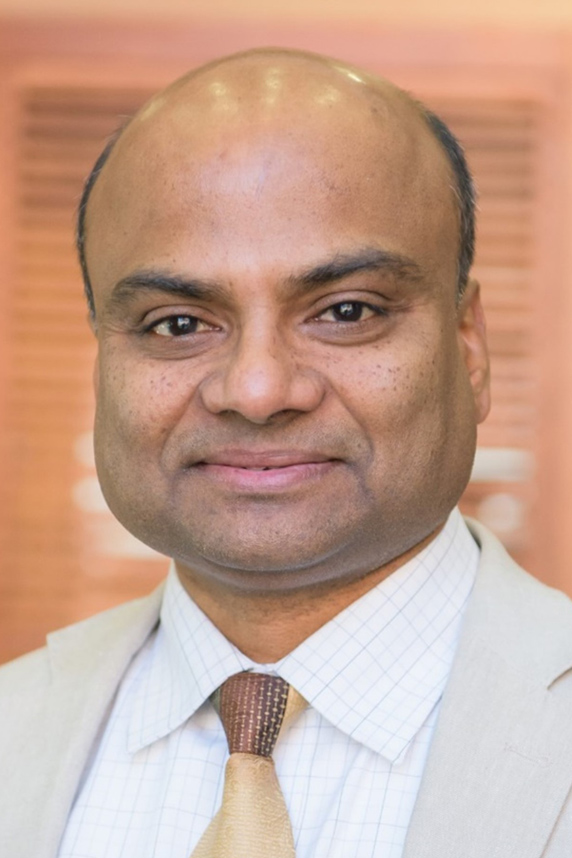
NIEHS grant recipient Upal Ghosh, Ph.D., is a professor of environmental engineering at the University of Maryland, Baltimore County, who has developed practical, scalable solutions to some of the toughest contamination problems we face. Much of his work focuses on reducing the bioavailability of pollutants — preventing them from entering the food web and reaching humans — in bodies of water such as lakes and rivers. SediMite, a technology he helped to create and commercialize, offers a sustainable solution to environmental cleanup of PCBs.
Beyond his efforts in the lab, Dr. Ghosh works closely with communities disproportionately affected by contamination. His projects in places like Washington, D.C., Baltimore, and Delaware have demonstrated the benefits of his remediation technology, helping to reduce fish consumption advisories, restore wetlands, and strengthen resiliency among residents.
In our conversation, Dr. Ghosh shared insights into the scale of environmental contamination globally and the evolving landscape of remediation technologies. He discussed the importance of understanding how exposures in a water body can lead to exposures in humans, as that knowledge is crucial to developing effective cleanup approaches. Dr. Ghosh also described his early interest in science and what inspired him to pursue a research career.
Economy and environment
Rick Woychik: Thank you, Dr. Ghosh, for taking the time to speak with me. Can you provide an overview of the scale of environmental contamination problems, both in the U.S. and globally?
Upal Ghosh: Sure. The way I think about it, the scale of environmental problems is related to the scale of the economy. A lot of the problems we discuss — from Superfund sites such as Love Canal to current challenges around PFAS contamination — are tied to how productive an economy is, and how production and usage practices have caused leakages.
The U.S. was the largest manufacturing center for many products, and we’re seeing the footprint of that. Love Canal is a prime example. All of these Superfund sites are remnants of an era where laws weren’t in place to internalize costs and reduce externalities, and now we’re realizing that.

I also travel internationally, and I see the same trends in developing countries. In India, they're ramping up production, and China has already reached high levels of industrial production. They’re starting to face challenges associated with manufacturing, transporting, and using large volumes of chemicals to provide their populations with a quality of life they expect and deserve.
In pharmaceutical production, we’re seeing leakages of pharmaceuticals, and with large-volume chemicals like petroleum and pesticides, every functioning economy creates this footprint. So, in short, the scale of the problem has often been tied to the scale of the economy. But I believe that new technologies will help to create a better dynamic, allowing for both strong economic growth and strong environmental health.
To solve the problem, first define it
RW: How do you go about developing remediation approaches?
UG: Environmental contamination poses an interesting challenge for scientists and engineers because the problem is often poorly defined. If I put PCB molecules in a beaker of clean water, as a scientist, I can describe the behavior accurately. But when I deal with a river, lake, or coastal bay, the matrix is much more complicated. For a variety of reasons, such as complex geochemistry, hydrodynamics, air-water exchange, and so forth, the attributes of contamination are not uniform. The behavior of compounds in that matrix becomes much more difficult to describe.
For example, in the PCB cases I’ve worked on, we have to try to understand not just what’s happening in a complex body of water, but also a complex ecological system that ultimately leads to human exposure via the food web. So, defining the problem is the first part of effective technology development, and in fact I think that’s where many technology needs lie: accurately defining exposure. We can’t solve the problem unless we do so.
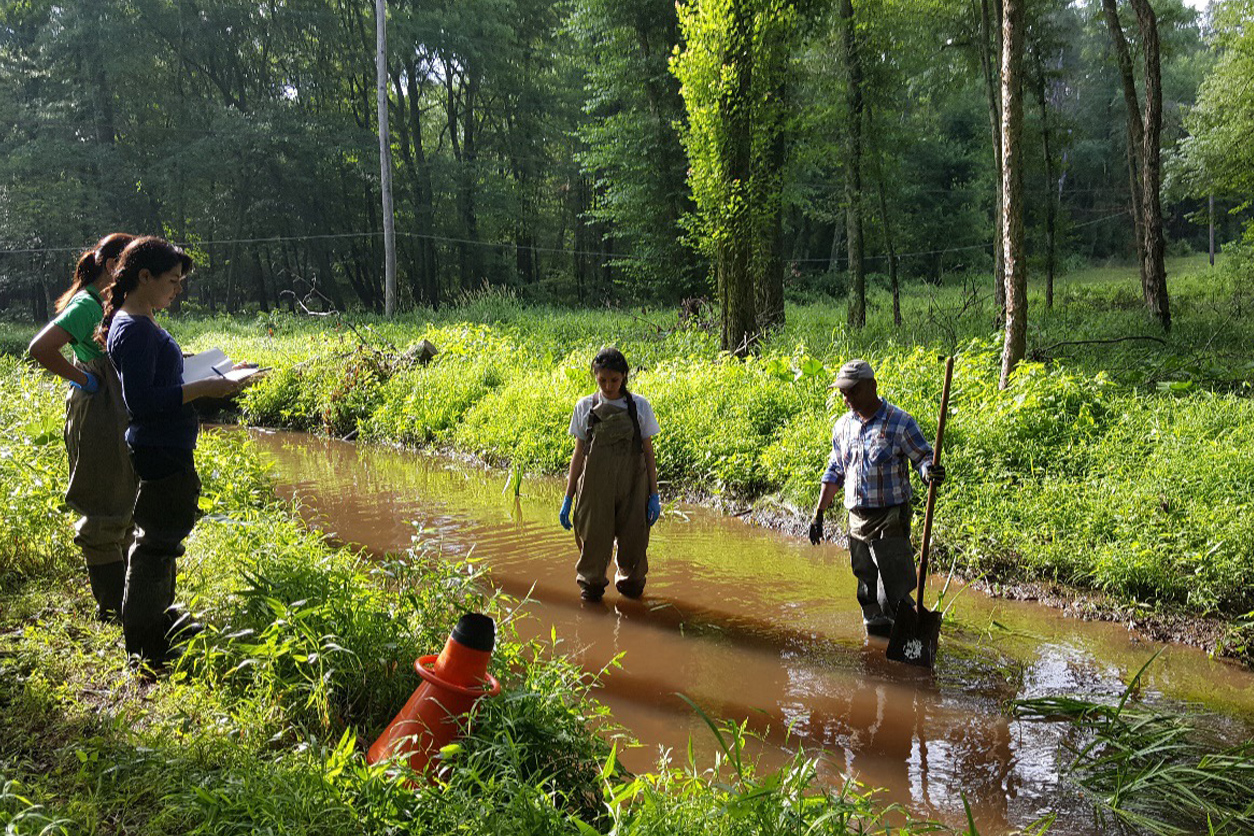
A lot of my work, and much of my colleagues’ work, falls under this category of accurately defining exposure, of understanding the bioavailability of pollutants in the soil or sediment environment. Once we understand the exposure — and the dominant exposure pathways — then I can bring in engineers, communities, and policymakers to create effective solutions.
Discovering black carbon’s key role
RW: This is very interesting. If I’m understanding you correctly, the goal is to not just determine PCB levels in the soil of a riverbed, for example, but also to assess how much PCB in the soil of the riverbed will make it into the water and be ingested by fish, or absorbed through their gills, and eventually consumed by humans. Is that what you’re getting at?
UG: That’s exactly right. It’s not just about measuring contamination in sediments but understanding how it moves through the aquatic environment and enters the food web. Once we can accurately define the dominant exposure pathways, we can tailor our remediation efforts to address the most critical sources of exposure. To that end, we’ve adopted passive sampling techniques and environmental modeling methods to better assess the movement and exposures.
Early in my research, when I was at Stanford working with my colleague and mentor Dr. Dick Luthy, we were studying the bioavailability of pollutants, looking specifically at PAHs [polycyclic aromatic hydrocarbons] and PCBs in sediments. The question we were asking was: Why is the bioavailability so different across sites? For example, when I took sediments from the Baltimore harbor versus sediments from the Milwaukee harbor, they didn’t behave the same.
Just looking at the organic matter content, mineral content, and particle size wasn’t explaining the differences, which were sometimes more than an order of magnitude. In some sediments, pollutants like PAHs and PCBs were bound up much more strongly than we would predict. So, we started looking at that more carefully and discovered that in sediments where pollutants were strongly bound and less bioavailable, black carbon was present. Black carbon, the graphitic form of carbon, occurs naturally and can also come from things like forest fires, coal coke, and soot.
We showed that these naturally present black carbon particles were binding PAHs and PCBs with affinities two orders of magnitude stronger than organic materials of plant origin. That was interesting because we could now explain the difference across our study sites. Some of our early papers focused on these natural differences in organic matter geochemistry, explaining the differences in bioavailability. Of course, we didn’t stop at just understanding the science — we wanted to use that knowledge to develop remediation technology.
Pellets pack a punch
RW: And this is where the product you helped to develop, SediMite, comes into play. Can you explain the technology behind it?
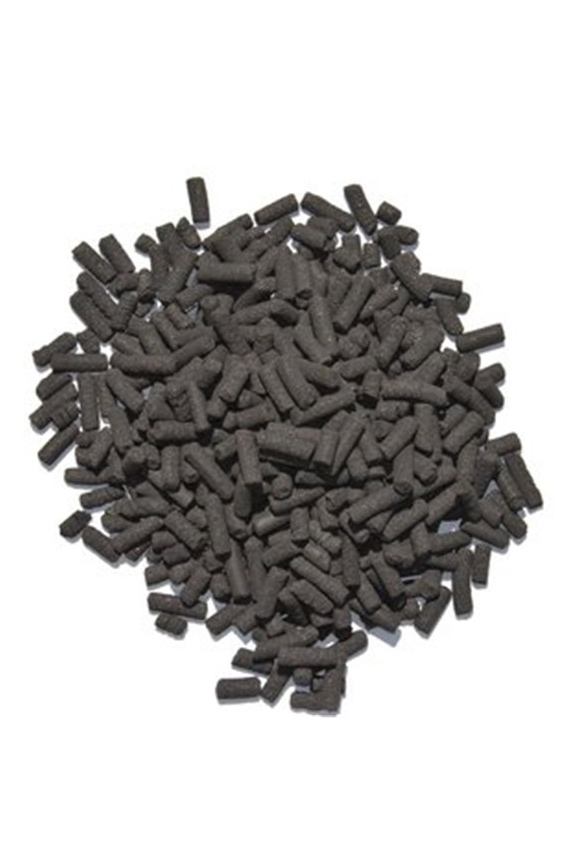
UG: SediMite works by binding contaminants in sediments, reducing their availability to organisms in the food web. The product consists of activated carbon packaged into pellets, which makes it easy to handle and apply in the field. Once dispersed in sediments, the activated carbon binds to hydrophobic pollutants like PCBs, making them less bioavailable to aquatic organisms. It can apply to a whole range of hydrophobic chemicals — pesticides, dioxins, and even PFAS. It works for some metals, too. We have done some work with mercury, and it binds strongly.
What sets this technology apart from traditional methods like dredging is that it minimizes environmental disruption. Dredging can release buried contaminants into the water, potentially exacerbating the problem. With SediMite, we’re able to stabilize contaminants in place, reducing the risk of exposure without disturbing the ecosystem. Additionally, this method is often more cost-effective than large-scale dredging and landfill disposal. SediMite can also be adjusted, so we can blend new formulations of absorbents in our pellets to target different pollutants.
Targeting contamination hotspots
RW: It sounds like this technology has the potential to significantly improve remediation efforts. How scalable is it? Could it be applied to larger bodies of water, like Lake Michigan?
UG: Scaling is always a consideration, and while SediMite can be successfully applied to larger areas, it’s often most effective in targeted locations where contamination levels are highest. With targeted dispersal, we have been able to reduce PCB bioavailability by 80% or more. We’ve successfully applied this technology in places like Mirror Lake in Delaware, where it helped to reduce concentrations in fish to levels below consumption advisory guidelines.
The broader notion of introducing activated carbon in a contaminated site, demonstrated successfully through our research, has now become mainstream technology. For example, the technique has been applied at multiple Superfund sites, such as the Passaic River in New Jersey.
In a large body of water like Lake Michigan, the focus would likely be on contaminated hotspots near industrial sites rather than attempting to treat the entire lake. This targeted approach allows for more efficient use of resources while still achieving significant risk reduction.
Recently, the U.S. Environmental Protection Agency used our product in Minnesota’s Scanlon Reservoir to clean up dioxins, and they covered about 15 acres. They used SediMite to reduce bioavailability in shoreline areas with wetlands without destroying the wetlands.
Using microbes to degrade contaminants
RW: One intriguing aspect of your work involves microbial degradation of contaminants. Can you tell us more about that?
UG: Absolutely. Microbial degradation is the ultimate solution — finding ways to not only bind contaminants but also break them down over time. In the case of PCBs, there are naturally occurring microorganisms that can dechlorinate these compounds, making them less harmful. We’ve worked with my microbiologist colleague Dr. Kevin Sowers to isolate these organisms, grow them in the lab, and then reintroduce them into contaminated environments as microbial catalysts. This process enhances the natural degradation of contaminants, further reducing their impact over time.
For the benefit of communities
RW: It’s inspiring to see remediation technologies applied in the real world. Could you share some examples of how your work has benefited communities disproportionately affected by environmental contamination?
UG: One project that stands out is the Middle Branch Resiliency Initiative in Southwest Baltimore, a community that faces multiple environmental challenges, including contaminated sediments and coastal flooding. By using SediMite to treat nearby sediments and creating elevated wetlands, we’re not only improving environmental conditions but also helping to protect the community from future flooding events. This project shows how environmental remediation can be integrated with broader resilience and restoration efforts, ultimately enhancing the quality of life for residents.
(Rick Woychik, Ph.D., directs NIEHS and the National Toxicology Program.)